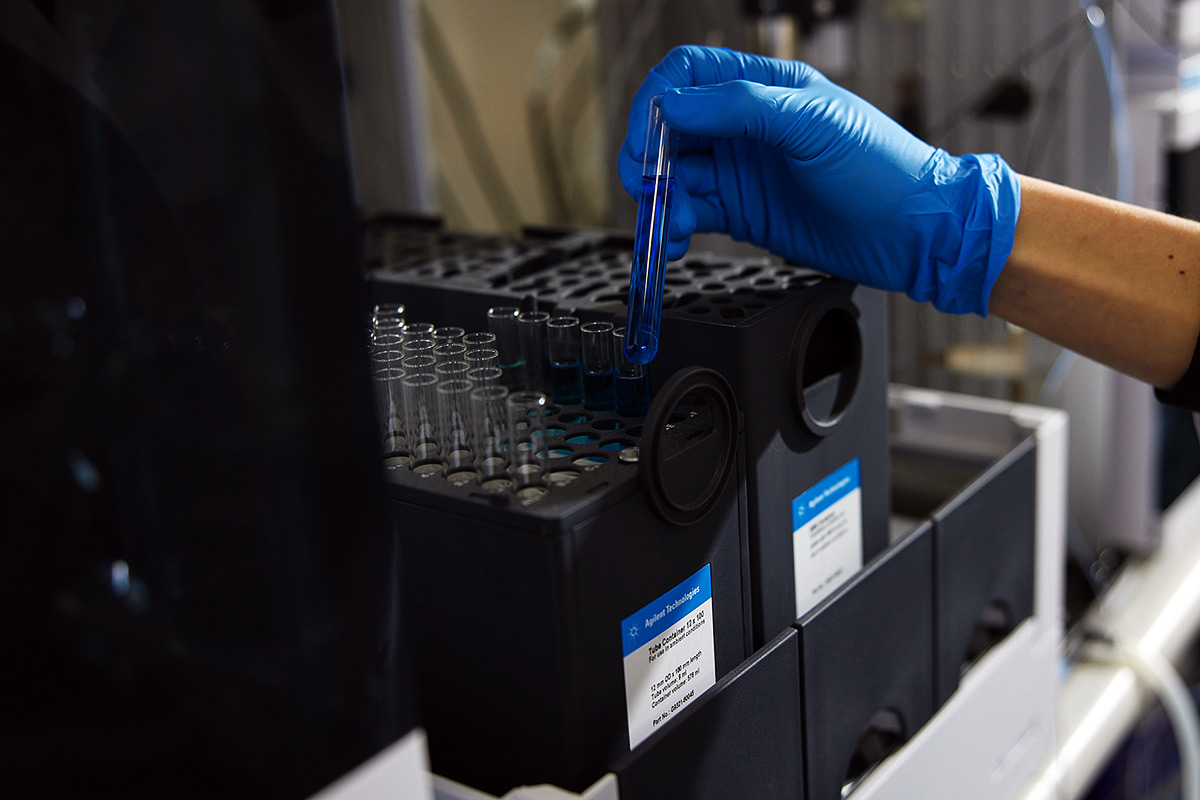
Research
Our dynamic research program explores the state-of-the-art in mRNA and LNP science and takes it further.
mRNA production
mRNA synthesis
Messenger RNA (mRNA) is a single stranded nucleic acid which is translated in the cell cytoplasm to produce the protein coded into its sequence. 1 While our bodies naturally produce mRNA, we can synthesise mRNA to code for a specific protein of interest. The initial step for mRNA synthesis is the design and development of a circular DNA (cDNA) template.2 This template is comprised of:
-
5’ and 3’ UTR: UTRs (untranslated regions) are non-coding sequences that are crucial for translation, stability and subcellular localisation of mRNA. The 5’ UTR is associated with the initiation of translation, while the 3’ UTR increases mRNA stability and gene expression through improved stabilisation. It also regulates mRNA translation efficiency.
-
ORF: The ORF (open reading frame), also known as the coding region, encodes the sequence of the protein of interest. This can be optimised through codon selection, placement and linear design, to enhance protein expression without affecting the encoded sequence. It usually starts with a start codon (AUG) and ends with a stop codon (UAA, UAG, UGA).
-
T7 Promoter: The T7 promoter sequence, which is recognised by an enzyme called T7 RNA polymerase, is vital to initiate the synthesis of mRNA from the cDNA template. 3
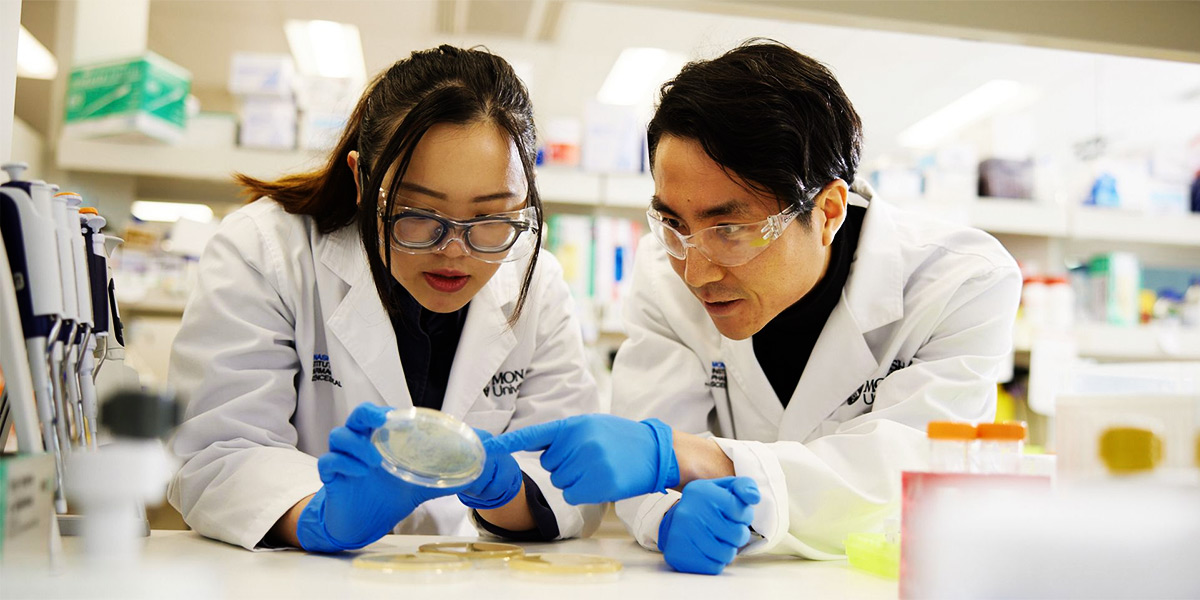
The cDNA is linearised using restriction digestion enzymes, and then amplified using a process called PCR (polymerase chain reaction). The poly(A) tail of the mRNA is also generated in this reaction.
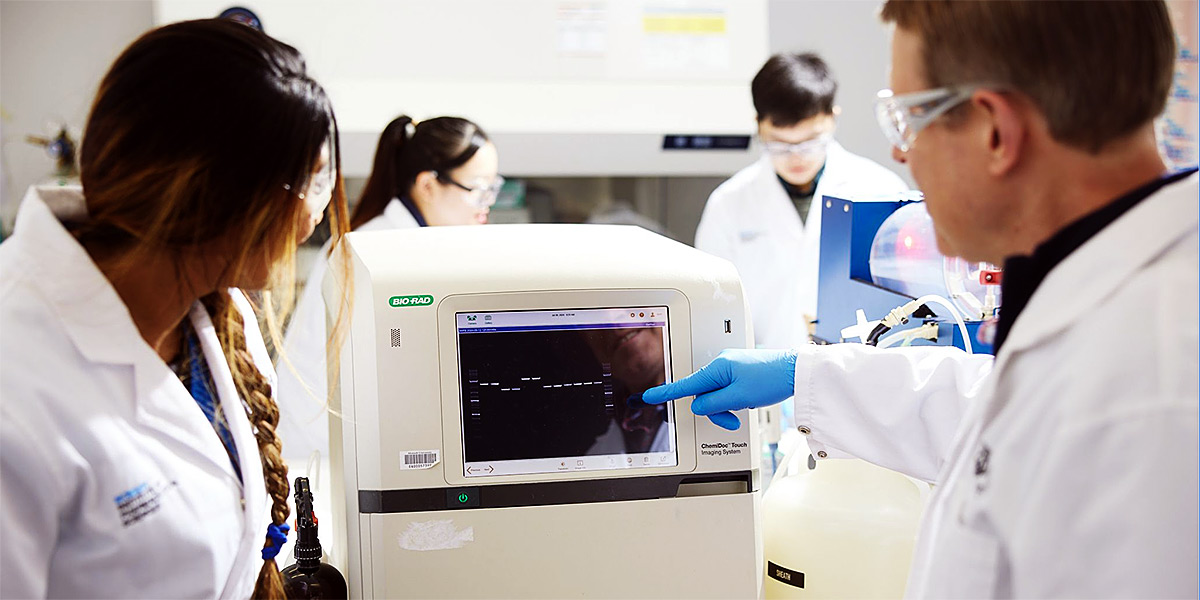
-
Poly(A) tail: This is 100-150 units of the nucleotide adenine placed at the 3’ end. It protects mRNA from enzymatic degradation, improves stability, and also has a role in the initiation of mRNA translation.
-
5’ Cap: Found in eukaryotic cells, this is essential to protect the mRNA from degradation and for efficient mRNA translation.4 Capping reagents used in mRNA products include Clean Cap AG, Clean Cap AG (3’ OMe) and Clean Cap GG.
-
Addition of modified nucleotides: Although using natural amino acids have its benefits, modified nucleotides have been found to improve mRNA translation while reducing undesirable immune responses. Typically, the nucleotide uracil (U) is replaced by a synthetic alternative. Modified nucleotides commonly used in synthetic mRNA products include N1-methyl pseudouridine (me1Ψ), pseudouridine (Ψ) and 5-methoxyuridine (5moU).5,6
The amplified cDNA template is then ready to use for mRNA synthesis. The clean Cap is commonly introduced during this step, together with the nucleotides needed to build the mRNA sequence and the T7 RNA polymerase.
The synthesised mRNA is purified with DNAse treatment to remove any leftover DNA. This is followed by a purification process to get rid of any impurities and leftover reagents, generally through precipitation with lithium chloride. Finally, any double-stranded RNA made during mRNA synthesis is removed: typical methods include cellulose, HPLC and oligo-DT purification. 7 The mRNA is then stored in an RNA storage solution at either physiological or acidic conditions at ultracold temperatures prior to use.
mRNA synthesis and sequence structure
Process of mRNA synthesis from a DNA template, with key elements of the sequence highlighted.
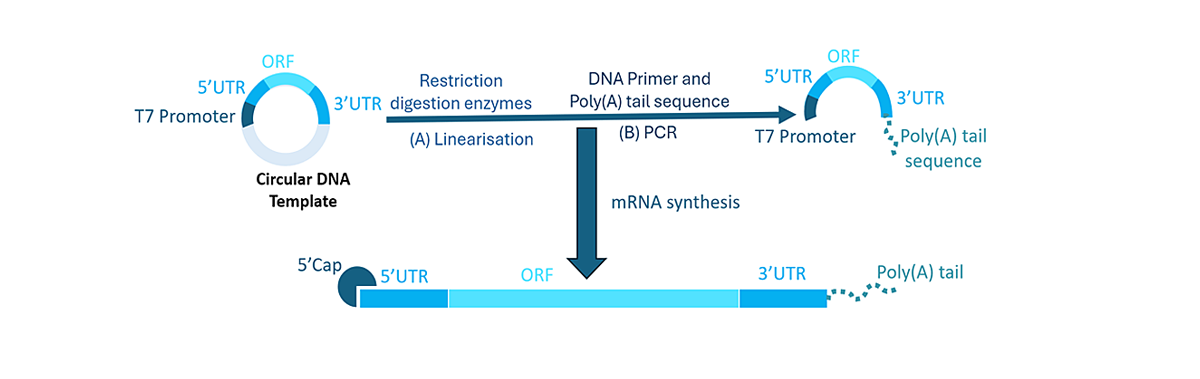
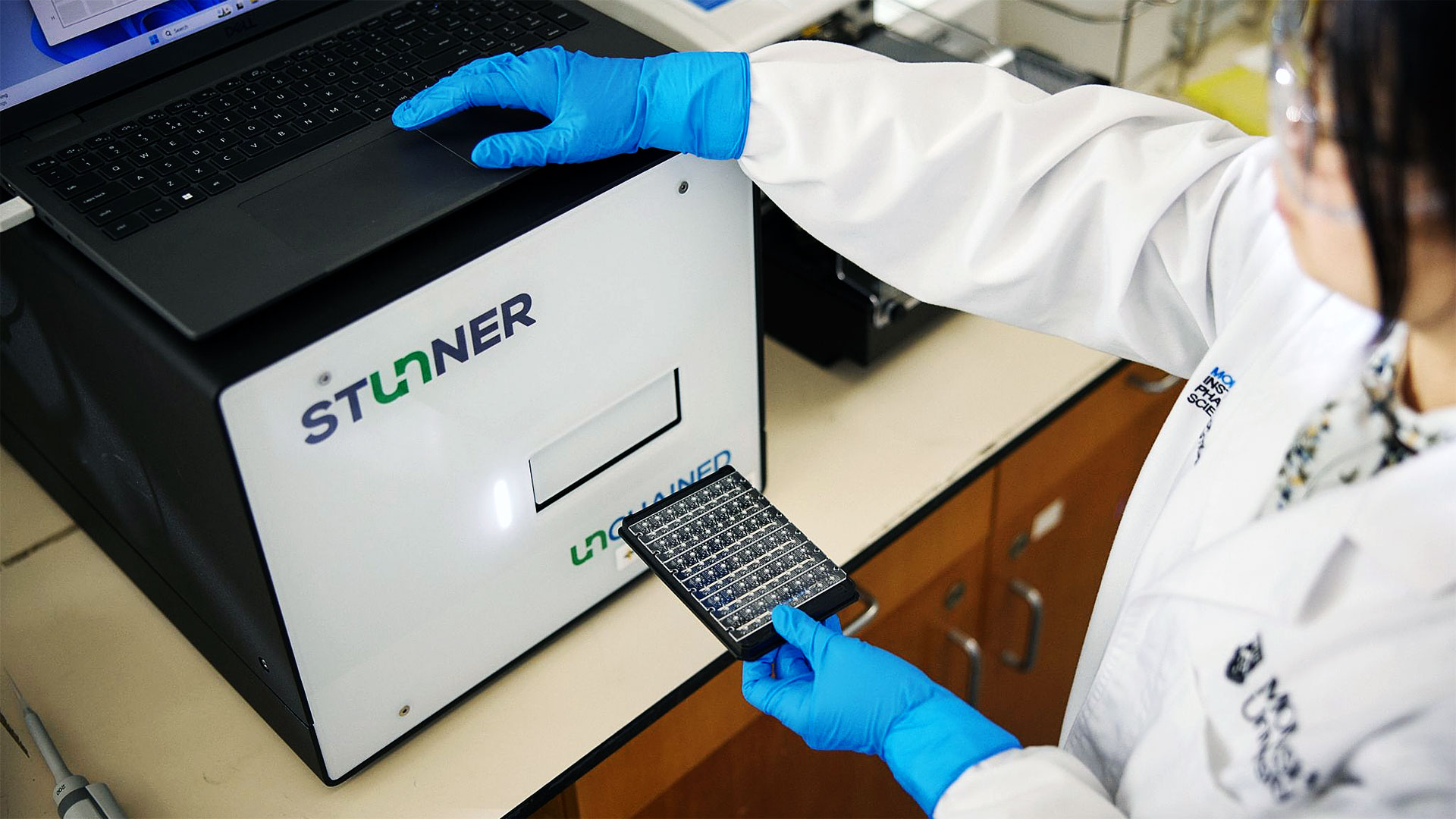
![]()
In partnership with the Monash Institute of Pharmaceutical Sciences and Therapeutic Innovation Australia, we provide multiple services across the mRNA space, utilising cutting edge technology and platforms – including Australia’s first rotating angle dynamic light scattering machine.
mRNA Research
Lipid nanoparticle formulation
The development of the lipid nanoparticle (LNP) platform has revolutionised RNA delivery.8 Previously, RNA therapies were stymied by two key problems: first, RNA is very susceptible to degradation and clearance by the body when delivered alone. Second: existing delivery platforms, such as liposomes, lacked effective mechanisms for the load and release of RNA. Optimisation of the now standard four-lipid LNP formulation during the 2010s9 allowed RNA to be encapsulated and delivered at high efficiency, and has since resulted in a number of RNA therapeutics progressing from the bench to the clinic – including the Moderna and Pfizer-BioNTech COVID-19 vaccines.
In addition to the mRNA cargo, a standard LNP formulation will include:
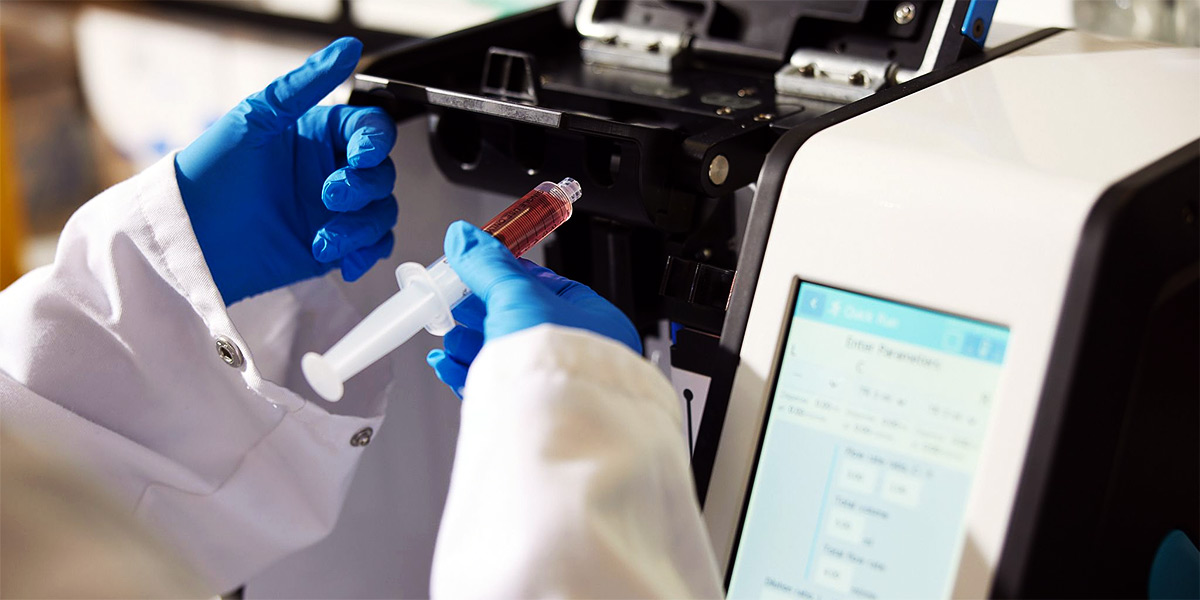
-
Ionisable lipid (~40-60%), which has no charge at pH 7, has the ability to become positively charged at acidic pH. This property is utilised to effectively encapsulate negatively charged mRNA into the particles, and also to release mRNA into the cell cytoplasm during the internalisation process (known as ‘endosomal escape’). Sometimes, cationic lipids, which have a permanent positive charge, are used for this purpose instead; however, as they have a higher risk of inducing cellular toxicity, generally ionisable lipids are preferred. There are a large variety of ionisable lipids being explored for LNP formulation.10 Ionisable lipids used in clinical formulations include DLin-MC3-DMA, SM-102, and ALC-0315.
-
Sterol lipid (~30-50%). These determine the fluidity of the particle and help maintain its structural integrity, in addition to aiding in membrane fusion of LNPs to a target cell. Though various sterol lipids have been investigated,11 the gold standard sterol lipid utilised in all current clinical formulations is cholesterol.
-
Phospholipid (~8-12%), also referred to as the ‘helper lipid’, is a key structural component of LNP formulations, and also contributes to membrane fusion of LNPs to a target cell. Typically the phospholipid component is neutrally charged, though zwitterionic phospholipids have also been explored.12 All clinical formulations currently use the lipid DSPC for this purpose.
-
PEG lipid, or PEGylated lipid (~0.25-2%) consists of a phospholipid conjugated to the polymer polyethylene glycol (PEG). PEG is known as a ‘stealth polymer’ for its ability to prevent proteins in the circulation from binding, thus helping hide LNPs from immune cells that might try to clear them before they reach their target. These lipids also have a role in controlling particle size and structure. Though a range of polymers are being explored as alternatives for LNP formulations,13 PEG remains the gold standard. PEG lipids used in clinical formulations include DMG-PEG2000 and ALC-0159.
While a number of methods can be used to form LNPs, typically lipids (in an organic phase) are mixed together with RNA (in an acidic aqueous phase) on a microfluidic platform utilising a herringbone or toroidal mixer.14 Partially-formed particles are then subjected to buffer exchange to remove the organic solvent and bring the solution to physiological pH. The final LNP structure that is formed is a spherical particle, typically around 100 nm in diameter; this is particularly preferred for vaccine applications, as it allows particles to access the lymphatic system.15 Optionally, a cryoprotectant such as sucrose will be added, to allow LNPs to be stored at ultracold temperatures and maintain their structural integrity.
mRNA-LNP formulation
Process of encapsulation of mRNA within lipid nanoparticles using toroidal mixing.
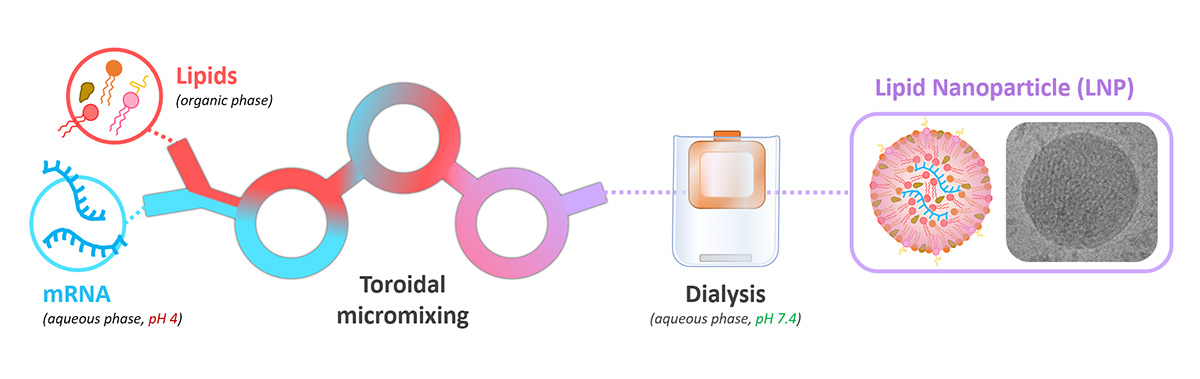
mRNA Research
References
- N. Chaudhary et al., Nat. Rev. Drug Discov. 2021, doi: 10.1038/s41573-021-00283-5
- H. Kwon et al., Biomaterials 2018, doi: 10.1016/j.biomaterials.2017
- A. D. Perenkov et al., Vaccines (Basel) 2023, doi: 10.3390/vaccines11101600
- M. Inagaki et al., Nat. Commun. 2023, doi: 10.1038/s41467-023-38244-8
- K. Karikó et al., Mol. Ther. 2008, doi: 10.1038/mt.2008.200
- K. Q. Kim et al., Cell Rep. 2022, doi: 10.1016/j.celrep.2022.111300
- V. Gote et al., Int. J. Mol. Sci. 2023, doi: 10.3390/ijms24032700
- E. H. Pilkington et al., Acta Biomater. 2021, doi: 10.1016/j.actbio.2021.06.023
- M. Jayaraman et al., Angew. Chem. 2012, doi: 10.1002/ange.201203263
- X. Han et al., Nat. Commun. 2021, doi: 10.1038/s41467-021-27493-0
- S. Patel et al., Nat. Commun. 2020, doi: 10.1038/s41467-020-14527-2
- S. Liu et al. Nat. Mater. 2021, doi: 10.1038/s41563-020-00886-0
- D. N. B. P. Hassanel et al. Int. J. Pharm. 2024, doi: 10.1016/j.ijpharm.2024.124695
- G. Prakash et al., Adv. Drug Deliv. Rev. 2022, doi: 10.1016/j.addr.2022.114197
- N. Trevaskis et al., Nat. Rev. Drug Discov. 2015, doi: 10.1038/nrd4608